How Batteries Work
What's actually going on inside your smartphone battery right now? The answer, it turns out, is: rather a lot.
One of the first things I set out to do before writing Material World was to try to get my head around the basics of how batteries work.
Much ink is spilled these days about these little cells and their Importance to the modern world (and the modern economy). But it is one thing to drone on about something being Very Important; it is quite another to understand why. And in all the thousands of wise words I had read about batteries in wise newspapers, I had encountered precious little to explain how they actually worked.
Well, I’m happy to say I now have at least a rudimentary grasp and, after reading this piece, so will you. If you read Material World you will understand even more. And what you’ll hopefully find, as I did, is that once you grasp what’s going on inside a battery, suddenly a lot of other things begin to make sense too: why do we need so many exotic minerals for batteries, why do they have a tendency to go up in flames, why are they so expensive, why, in short, do so many facets of modern life - getting to net zero, getting the right Brexit deal and so on - come back to batteries?
So I’ve written the below as a kind of primer, which might help fill in some gaps amid all the abstract talk in the news these days about cells and gigafactories and lithium.
What batteries do and don’t do
The first thing to note is the most blindingly obvious: batteries aren’t a source of energy but a store of energy. The battery is really a means of converting electrical energy into electrochemical energy and back again, and the main chemical of choice we use for that these days is lithium.
Nearly every advanced type of battery you’ll encounter these days, whether it’s in your smartphone or indeed an electric car, is based on a lithium chemistry. There are other elements and compounds which have decent electrochemical potential: sodium is pretty good too, and since it’s significantly easier to get hold of than lithium a lot of people are talking about sodium ion batteries these days. But sodium, good as it is, is simply not as attractive, from an energy density perspective, as lithium. There’s no escaping the periodic table, and there is no other element quite as good for making batteries as lithium.
But having lots of electrochemical potential has its drawbacks too. And if you were ever shown pure lithium (or for that matter pure sodium) in chemistry class at school you’ll immediately understand one of them. Lithium is very, very volatile. Exposed to air or water it bangs and flames and explodes. It’s incredibly hard to handle.
And that’s the main reason it took ages for anyone to develop a working lithium battery. There’s an important lesson lurking here. Scientists were well aware for more than a century that lithium was the most promising battery material of all. But turning good ideas into actual physical products is often really hard.
This is one of the recurrent lessons you’ll encounter when you read Material World. How to make concrete? How to make a solid state switch (a transistor)? How to turn salt into critical chemicals? These were not questions that were answered in one Eureka moment. They were long-standing challenges that took decades of work, theory and experimentation to resolve. This is worth remembering every time you hear someone say this or that scientific advance will never happen because it’s always been a few decades away. Often these things do indeed take decades - sometimes centuries. But that doesn’t mean they won’t eventually happen.
Anyway, the challenge of making a lithium battery was eventually solved in the 1970s and ‘80s by a group of scientists. Three of them won the 2019 Nobel Prize for Chemistry and while they certainly deserve much of the praise, this was an enterprise on which hundreds if not thousands of scientists and manufacturers were engaged. The advances are still happening today.
What’s actually inside a battery?
But the main principle is now pretty much set in stone. The best way to get your head around it is to consider what you find inside a cylindrical cell - the kind of thing that looks a little like those AA cells you probably have some of in a drawer at home (albeit a bit bigger).
I used to think of these cells as quite literal cylinders, containing a kind of liquid fuel - probably because of childhood memories of when AA batteries would occasionally leak electrolyte out into my walkman. But actually it’s far more helpful to think of the internals of this battery as something else: a Swiss roll (or as they call them in the US, a “jelly roll”).
If you were to open up a cylindrical lithium ion battery (don’t actually do this - it’s very dangerous) what you would find inside is a long rolled up coil of thin sheets. Uncoil them entirely and what you’re left with are two long sheets of foil, perhaps a metre or so long, both covered with a kind of grey/black coating. They both look pretty unexceptional, but don’t be fooled because this is where much of the magic happens. These coiled-up foils are the cathode and anode. They are the cell’s electrodes, connected, usually via some copper tabs, to the positive and negative ends of the battery.
There’s a fairly easy way to tell which one is which: the cathode is generally a sheet of aluminium foil covered in a black substance. The anode is a copper foil coated with a black-grey substance. But ignore the metallic foil for the time being: it’s those dull, black substances we’re most interested in, because that’s where the clever chemicals are to be found.
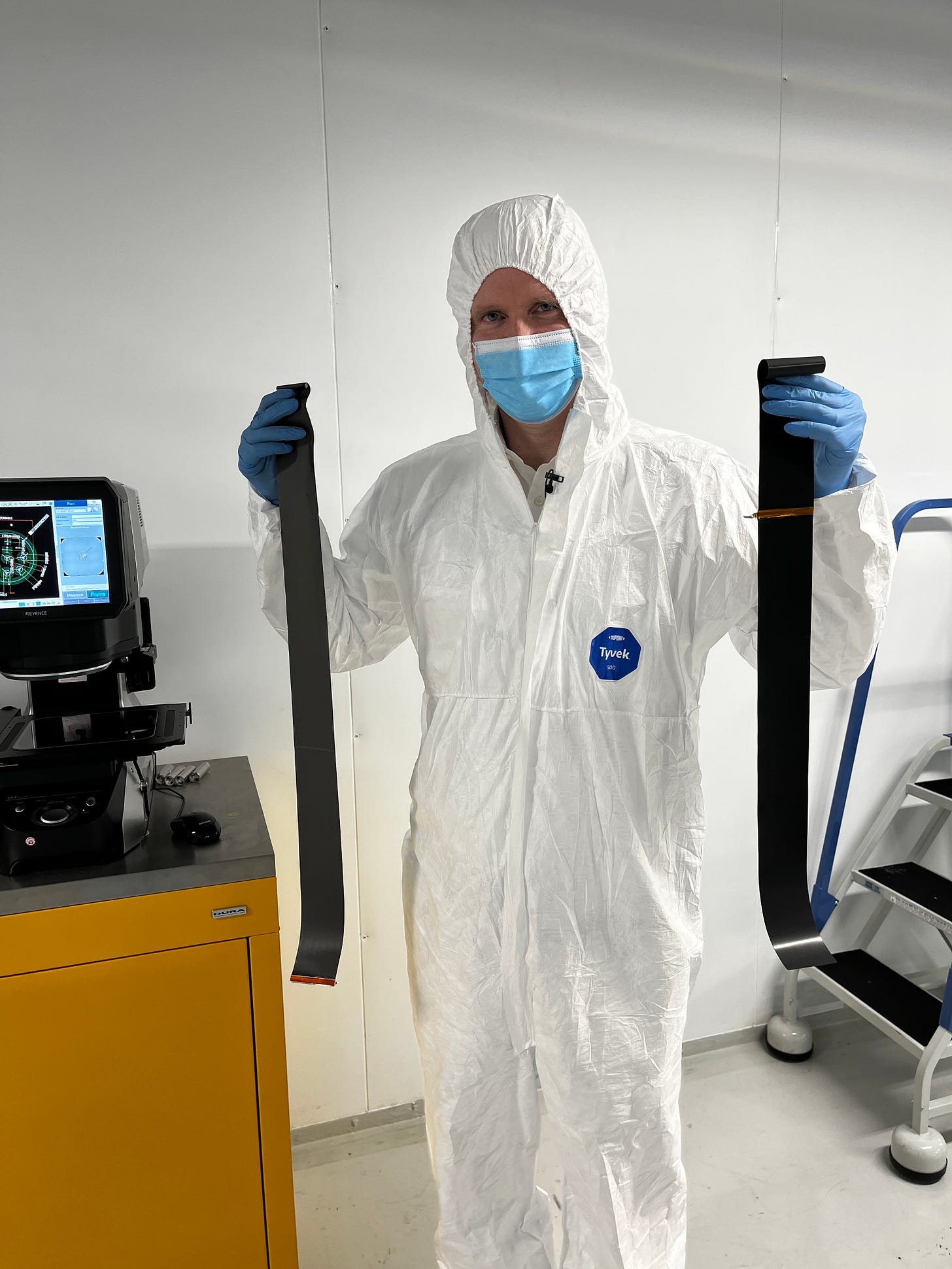
The cathode is the most important electrode - the one that costs the most, primarily because of the costly chemicals pasted onto it. This is where you’ll find much of the lithium in the battery. Though alongside the lithium are a host of even more exotic chemicals which help it do the kinds of things batteries have to do.
The most famous/infamous is cobalt, which you find in many battery chemistries, including most smartphone batteries. The highest performance car batteries tend to have lots of nickel in them, as well as cobalt and manganese. One way of conceptualising it is that these added chemicals are a kind of seasoning added to the main dish, spices that help the lithium to fulfil its main function of turning that electrical energy into chemical energy.
And there are many different potential recipes. This is not the place to get into the weeds of cathode chemistry, except to say a couple of things. First, the manufacture of these speciality chemicals is an incredibly complex business - a whole industrial sector of its own. Most battery companies actually buy in their cathode (and for that matter anode) chemicals and focus mainly on pasting them onto foil and coiling the foil into batteries.
Secondly, the ever changing nature of cathode chemistry is also having a bearing on some other relevant stories you might have encountered. You’re probably already aware that cobalt is one of the biggest challenges for the battery industry, because the vast majority of the metal is mined in the Democratic Republic of Congo, much of it in hideous conditions. What’s less widely realised is that while this is certainly still a big issue for battery producers, it’s not quite as big as it seemed a few years ago. In part that’s because we’ve gotten better at recycling cobalt but - even more importantly - it’s because these days there are electric car battery chemistries which exclude cobalt and do a pretty good job. Many of the electric cars you can buy today don’t have any cobalt in their batteries. On the flip side these so called lithium iron phosphate cells are mostly made in China so some would say we’re swapping one challenge with another. Anyway, much more on that in the book.
But back to the cathode in our Swiss roll: the key thing to remember is that this is where you’ll find most of the lithium. The anode, on the other hand, is pasted with a special kind of graphite. To be more precise, it’s a mixture of natural graphite, mined from the ground, and “synthetic” graphite, which is cooked up from crude oil. The precise ratio of synthetic to natural graphite varies. One rule of thumb I’ve heard is that natural graphite helps batteries keep their charge for longer while synthetic graphite allows for faster-charging batteries. More on this in a long piece I wrote for Sky News last year.
By the way, the cathode and anode aren’t the only things you’ll find in a battery. There’s also a thin white polymer strip which is coiled up between those two foils, and the whole canister is filled with a liquid electrolyte solution, rich in lithium. So there’s a bit of liquid there too.
The rocking chair
But anyway, we’ve now reached the most important bit - the main principle in how these batteries work. To help you I’ve drawn a couple of basic diagrams.
The first one shows you what’s happening when a battery is charging. Lithium ions, which is to say positively-charged lithium atoms, travel across from the cathode to the anode, via the liquid electrolyte and through the separator. It’s worth internalising this for a moment because it’s probably the most important concept of all. The lithium ions are physically moving from one electrode to another, to nestle somewhere in amongst the carbon atoms in the graphite. It’s a migration.
When your battery is fully charged, that means the anode is chock-full of lithium ions. Look: you can see them there in the carbon matrix of the anode foil in the next diagram. Much - most, really - of battery chemistry is all about trying to maximise the speed and effectiveness of this migration.
Trying to create the best cathode chemistry to allow those lithium ions to pass across to the anode, making the best carbon matrix which will allow the easy ingress and residence of lithium - that’s the goal. And part of the reason why battery materials are so expensive isn’t just that lithium and cobalt etc are quite expensive; it’s that you need to put a lot of effort and investment into making the purest, finest cathode and anode mixtures to allow this migration to happen - back and forth, back and forth.
Because what’s happening when a battery is discharging, in other words when you’re out and about with your smartphone, is that the lithium ions are quietly shuttling back from the anode to the cathode. On the way they release the electrochemical energy which sends power to your device.
When you press the pedal on your electric car, the circuitry is ordering the anode to send even more lithium ions back across to the cathode. Everything comes back to the shuttling of lithium ions back and forth. In the early days of batteries this became known as the “rocking chair” concept. I use a different analogy in my book but the principle is much the same. Back and forth they go, invisibly pulsing with electrochemical activity.
It’s quite helpful (possibly also slightly unsettling) to remind yourself of this to-and-fro motion the next time you fast charge your phone and notice it’s slightly warm to touch. That warmth is a consequence of the movement of these ions somewhere beneath the surface as they shift across and ever so slightly swell up the anode in the process.
Different on the outside, similar on the inside
It’s probably worth mentioning at this stage that the battery in your phone is not cylindrical like the ones I’ve described thus far, but, mostly likely, a small rectangular pouch. But the principle is much the same. Rather than being coiled together into a cylinder, the cathode and anode in a pouch cell are instead layered on top of each other. There are prismatic cells too. But I wouldn’t get too fixated with the dimensions of a battery. At heart they are all just confections of metallic foil coated with precious chemicals and nestled alongside plastic separators, bathed in a liquid electrolyte.
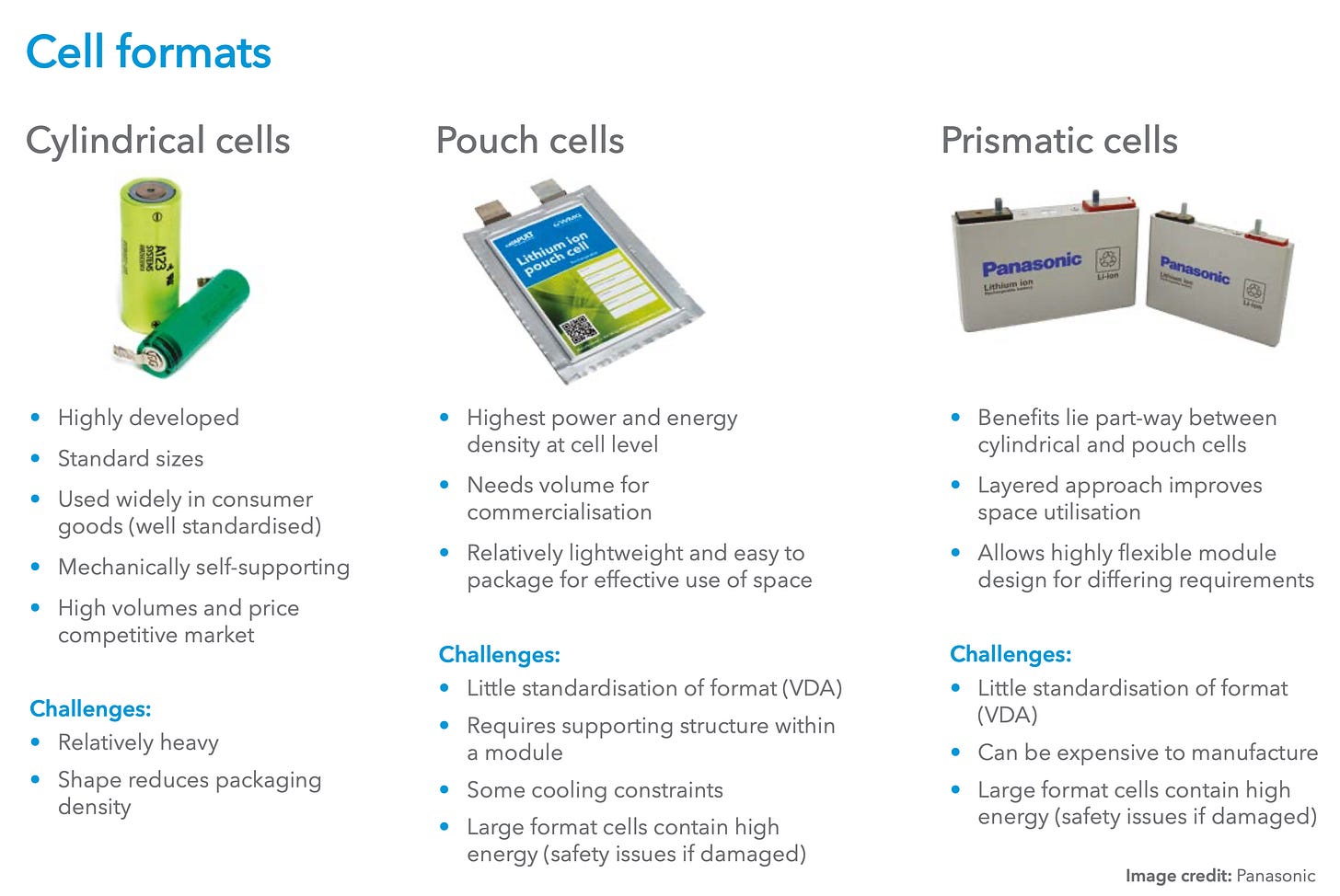
And that’s it. Batteries are little Swiss rolls and sandwiches of cathode and anode materials, carefully arrayed inside a package. There’s a special skill in assembling batteries, but there’s similarly a special skill in making the materials that get pasted onto those electrodes. There’s a magic to making the right separator which can allow lithium ions to pass freely from one side to another without allowing negative and positive electrodes to touch. There’s a whole chemical industry devoted to making the perfect electrolyte in which to bathe the solid bits of the battery.
As with so much in the Material World, the deeper one delves into the detail, the more fascinating it gets. And the more you understand these nuts and bolts the more you realise why it matters where we get this stuff. The race to build billions of these cells doesn’t just mean we need more lithium.
It also means we need much more nickel, manganese and cobalt. It means we need more separators, made, usually from petrochemicals derived from oil. It means we need more graphite, much of which also comes from crude. It means we need more chemicals plants turning out electrolytes. It’s a whole enormous supply chain - and much of that supply chain simply doesn’t exist in Europe right now.
I hope the above made some sense and was helpful. Do let me know via the comments if you’d like more along these lines. And (sorry - one more plug) there’s much more on the hidden sub-surface world of batteries (and the lengths we’re going to to get the materials we put inside them) in Material World. Do pre-order!
It certainly is fascinating!
At a half-step deeper, not all of the lithium in a battery moves. Some of it has to stay in the cathode to stop it collapsing. Much research is happening to increase the mobilisation fraction (while keeping the battery working). That would cut the amount of lithium required for a given capacity, and therefore cut the cost of the battery in a car.
Other research is about incorporating silicon into the anode alongside or replacing graphite to increase charging speed. Silicon brings its own problems to solve, though.
Yet other research is into improved electrolytes that would allow the anode to be metallic lithium, doing away with graphite altogether, saving weight and cost. This is one area in which AI might help, checking billions of chemical formulas for the right properties.
In line with the theme of this blog, it's lots of little changes, painstakingly researched over decades, that eventually add up to a big improvement in cost, weight, and durability. Eventually? No, rapidly, in the case of lithium batteries, compared to say lead-acid batteries or car paints.
Thanks book preordered; I am looking forward to it. Your insights into supply chains from raw materials, through intermediate processing, to final products - who, when where and how in elegant prose is educational and important.